작업기억
package.lua 80번째 줄에서 Lua 오류: module 'Module:Namespace detect/data' not found. 작업기억(Working memory)은 작업에 필요한 정보를 일시적으로 보유하는 제한된 용량을 가지고 있는 인지체계이다.[1] 작업기억은 추론과 결정, 행동의 안내에 있어 중요한 역할을 한다.[2][3] 작업기억은 대개 단기기억과 동의어로 사용되지만, 어떤 이론가들은 이 두 유형의 기억을 구별하여, 작업기억은 저장된 정보의 조작을 허용하는 반면 단기기억은 정보를 잠깐 저장할 뿐이라고 여긴다.[2][4] 작업기억은 인지심리학, 신경심리학, 신경과학 이론의 핵심 개념이다.
역사[편집]
"작업기억working memory"이라는 용어는 밀러, 갈란터, 프리브람이 지어냈으며[5][6] 1960년대에 마음을 컴퓨터에 비유하는 이론의 맥락에서 사용되었다. 1968년에 앳킨슨과 쉬프린은[7] 용어를 그들의 "단기 저장short-term store"을 묘사하기 위해 사용했다. 오늘날 작업기억이라고 불리는 것은 이전에는 "short-term store"이나 Short-term memor, primary memory, immediate memory, operant memory, provisional memory 등으로 지칭되었다.[8] 단기 기억은 몇 초 간의 짧은 시간 동안 정보를 기억하는 능력이다. 오늘날 대부분의 이론가들은 작업기억의 개념을 단기 기억의 오래된 개념을 대체하거나 포함하는 데 사용하여, 단순한 정보의 유지보다 정보의 조작의 개념을 더 강조한다.
20세기 초반에 힛지그와 페리어가 전전두엽 피질(PFC) 절제 실험에 대해 묘사했을 때 작업 기억의 신경적인 기반에 관한 실험이 처음으로 언급되었다. 그들은 전전두엽 피질이 감각적인 과정보다 인지적인 측면에 있어 좀 더 중요하다고 결론내렸다.[9] 칼라일 제이콥슨Carlyle Jacobsen과 동료들은 1935~36년에 전두엽 절제가 반응 지연에 어떻게 해로운 영향을 끼치는지에 대해 최초로 규명해냈다.[9][10]
이론[편집]
작업 기억이 해부학적으로, 인지적으로 어떻게 기능하는지에 대해서 수많은 모델들이 제시되었다. 이 중에서 제일 영향력있는 두 개의 모형을 밑에서 소개한다.
다성분 모델[편집]
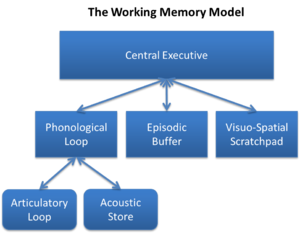
배들리와 히치는 1974년에[11] 작업 기억 다성분 모델을 도입했다. The theory proposed a model containing three components: the central executive, the phonological loop, and the visuospatial sketchpad with the central executive functioning as a control center of sorts, directing info between the phonological and visuospatial components.[12] The central executive is responsible inter alia for directing attention to relevant information, suppressing irrelevant information and inappropriate actions, and coordinating cognitive processes when more than one task is simultaneously performed. A "central executive" is responsible for supervising the integration of information and for coordinating "slave systems" that are responsible for the short-term maintenance of information. One slave system, the phonological loop (PL), stores phonological information (that is, the sound of language) and prevents its decay by continuously refreshing it in a rehearsal loop. It can, for example, maintain a seven-digit telephone number for as long as one repeats the number to oneself again and again.[13] The other slave system, the visuospatial sketchpad, stores visual and spatial information. It can be used, for example, for constructing and manipulating visual images and for representing mental maps. The sketchpad can be further broken down into a visual subsystem (dealing with such phenomena as shape, colour, and texture), and a spatial subsystem (dealing with location).
In 2000, Baddeley extended the model by adding a fourth component, the episodic buffer, which holds representations that integrate phonological, visual, and spatial information, and possibly information not covered by the slave systems (e.g., semantic information, musical information). The episodic buffer is also the link between working memory and long-term memory.[14] The component is episodic because it is assumed to bind information into a unitary episodic representation. The episodic buffer resembles Tulving's concept of episodic memory, but it differs in that the episodic buffer is a temporary store.[15]
장기기억의 일부분으로써의 작업기억[편집]
Anders Ericsson and Walter Kintsch[16] have introduced the notion of "long-term working memory", which they define as a set of "retrieval structures" in long-term memory that enable seamless access to the information relevant for everyday tasks. In this way, parts of long-term memory effectively function as working memory. In a similar vein, Cowan does not regard working memory as a separate system from long-term memory. Representations in working memory are a subset of representations in long-term memory. Working memory is organized into two embedded levels. The first consists of long-term memory representations that are activated. There can be many of these—there is theoretically no limit to the activation of representations in long-term memory. The second level is called the focus of attention. The focus is regarded as having a limited capacity and holds up to four of the activated representations.[17]
Oberauer has extended Cowan's model by adding a third component, a more narrow focus of attention that holds only one chunk at a time. The one-element focus is embedded in the four-element focus and serves to select a single chunk for processing. For example, four digits can be held in mind at the same time in Cowan's "focus of attention". When the individual wishes to perform a process on each of these digits—for example, adding the number two to each digit—separate processing is required for each digit since most individuals cannot perform several mathematical processes in parallel.[18] Oberauer's attentional component selects one of the digits for processing and then shifts the attentional focus to the next digit, continuing until all digits have been processed.[19]
용량[편집]
작업기억은 일반적으로 제한된 용량을 가지고 있다고 여겨진다. 단기 기억의 용량 한도에 대한 초기의 측정은 1956년에 밀러가 제안한 ""이었다. considered to have limited capacity. An early quantification of the capacity limit associated with short-term memory was the "magical number seven" suggested by Miller in 1956.[20] He claimed that the information-processing capacity of young adults is around seven elements, which he called "chunks", regardless of whether the elements are digits, letters, words, or other units. Later research revealed this number depends on the category of chunks used (e.g., span may be around seven for digits, six for letters, and five for words), and even on features of the chunks within a category. For instance, span is lower for long than short words. In general, memory span for verbal contents (digits, letters, words, etc.) depends on the phonological complexity of the content (i.e., the number of phonemes, the number of syllables),[21] and on the lexical status of the contents (whether the contents are words known to the person or not).[22] Several other factors affect a person's measured span, and therefore it is difficult to pin down the capacity of short-term or working memory to a number of chunks. Nonetheless, Cowan proposed that working memory has a capacity of about four chunks in young adults (and fewer in children and old adults).[23]
Whereas most adults can repeat about seven digits in correct order, some individuals have shown impressive enlargements of their digit span—up to 80 digits. This feat is possible by extensive training on an encoding strategy by which the digits in a list are grouped (usually in groups of three to five) and these groups are encoded as a single unit (a chunk). For this to succeed, participants must be able to recognize the groups as some known string of digits. One person studied by Ericsson and his colleagues, for example, used an extensive knowledge of racing times from the history of sports in the process of coding chunks: several such chunks could then be combined into a higher-order chunk, forming a hierarchy of chunks. In this way, only some chunks at the highest level of the hierarchy must be retained in working memory, and for retrieval the chunks are unpacked. That is, the chunks in working memory act as retrieval cues that point to the digits they contain. Practicing memory skills such as these does not expand working memory capacity proper: it is the capacity to transfer (and retrieve) information from long-term memory that is improved, according to Ericsson and Kintsch (1995; see also Gobet & Simon, 2000[24]).
Measures and correlates과 상호관련[편집]
Working memory capacity can be tested by a variety of tasks. A commonly used measure is a dual-task paradigm, combining a memory span measure with a concurrent processing task, sometimes referred to as "complex span". Daneman and Carpenter invented the first version of this kind of task, the "reading span", in 1980.[25] Subjects read a number of sentences (usually between two and six) and tried to remember the last word of each sentence. At the end of the list of sentences, they repeated back the words in their correct order. Other tasks that do not have this dual-task nature have also been shown to be good measures of working memory capacity.[26] Whereas Daneman and Carpenter believed that the combination of "storage" (maintenance) and processing is needed to measure working memory capacity, we know now that the capacity of working memory can be measured with short-term memory tasks that have no additional processing component.[27][28] Conversely, working memory capacity can also be measured with certain processing tasks that don't involve maintenance of information.[29][30] The question of what features a task must have to qualify as a good measure of working memory capacity is a topic of ongoing research.
Measures of working-memory capacity are strongly related to performance in other complex cognitive tasks, such as reading comprehension, problem solving, and with measures of intelligence quotient.[31]
Some researchers have argued[32] that working-memory capacity reflects the efficiency of executive functions, most notably the ability to maintain multiple task-relevant representations in the face of distracting irrelevant information; and that such tasks seem to reflect individual differences in the ability to focus and maintain attention, particularly when other events are serving to capture attention. Both working memory and executive functions rely strongly, though not exclusively, on frontal brain areas.[33]
Other researchers have argued that the capacity of working memory is better characterized as the ability to mentally form relations between elements, or to grasp relations in given information. This idea has been advanced, among others, by Graeme Halford, who illustrated it by our limited ability to understand statistical interactions between variables.[34] These authors asked people to compare written statements about the relations between several variables to graphs illustrating the same or a different relation, as in the following sentence: "If the cake is from France, then it has more sugar if it is made with chocolate than if it is made with cream, but if the cake is from Italy, then it has more sugar if it is made with cream than if it is made of chocolate". This statement describes a relation between three variables (country, ingredient, and amount of sugar), which is the maximum most individuals can understand. The capacity limit apparent here is obviously not a memory limit (all relevant information can be seen continuously) but a limit to how many relationships are discerned simultaneously.
Experimental studies of working-memory capacity작업 기억 용량에 대한 실증적 연구[편집]
There are several hypotheses about the nature of the capacity limit. One is that a limited pool of cognitive resources needed to keep representations active and thereby available for processing, and for carrying out processes.[35] Another hypothesis is that memory traces in working memory decay within a few seconds, unless refreshed through rehearsal, and because the speed of rehearsal is limited, we can maintain only a limited amount of information.[36] Yet another idea is that representations held in working memory interfere with each other.[37]
Decay theories감쇠 이론[편집]
The assumption that the contents of short-term or working memory decay over time, unless decay is prevented by rehearsal, goes back to the early days of experimental research on short-term memory.[38][39] It is also an important assumption in the multi-component theory of working memory.[40] The most elaborate decay-based theory of working memory to date is the "time-based resource sharing model".[41] This theory assumes that representations in working memory decay unless they are refreshed. Refreshing them requires an attentional mechanism that is also needed for any concurrent processing task. When there are small time intervals in which the processing task does not require attention, this time can be used to refresh memory traces. The theory therefore predicts that the amount of forgetting depends on the temporal density of attentional demands of the processing task—this density is called "cognitive load". The cognitive load depends on two variables, the rate at which the processing task requires individual steps to be carried out, and the duration of each step. For example, if the processing task consists of adding digits, then having to add another digit every half second places a higher cognitive load on the system than having to add another digit every two seconds. In a series of experiments, Barrouillet and colleagues have shown that memory for lists of letters depends neither on the number of processing steps nor the total time of processing but on cognitive load.[42]
Resource theories자원 이론[편집]
Resource theories assume that the capacity of working memory is a limited resource that must be shared between all representations that need to be maintained in working memory simultaneously.[43] Some resource theorists also assume that maintenance and concurrent processing share the same resource;[35] this can explain why maintenance is typically impaired by a concurrent processing demand. Resource theories have been very successful in explaining data from tests of working memory for simple visual features, such as colors or orientations of bars. An ongoing debate is whether the resource is a continuous quantity that can be subdivided among any number of items in working memory, or whether it consists of a small number of discrete "slots", each of which can be assigned to one memory item, so that only a limited number of about 3 items can be maintained in working memory at all.[44]
Interference theories방해 이론[편집]
Several forms of interference have been discussed by theorists. One of the oldest ideas is that new items simply replace older ones in working memory. Another form of interference is retrieval competition. For example, when the task is to remember a list of 7 words in their order, we need to start recall with the first word. While trying to retrieve the first word, the second word, which is represented in proximity, is accidentally retrieved as well, and the two compete for being recalled. Errors in serial recall tasks are often confusions of neighboring items on a memory list (so-called transpositions), showing that retrieval competition plays a role in limiting our ability to recall lists in order, and probably also in other working memory tasks. A third form of interference is the distortion of representations by superposition: When multiple representations are added on top of each other, each of them is blurred by the presence of all the others.[45] A fourth form of interference assumed by some authors is feature overwriting.[46][47] The idea is that each word, digit, or other item in working memory is represented as a bundle of features, and when two items share some features, one of them steals the features from the other. The more items are held in working memory, and the more their features overlap, the more each of them will be degraded by the loss of some features.
Limitations한계[편집]
None of these hypotheses can explain the experimental data entirely. The resource hypothesis, for example, was meant to explain the trade-off between maintenance and processing: The more information must be maintained in working memory, the slower and more error prone concurrent processes become, and with a higher demand on concurrent processing memory suffers. This trade-off has been investigated by tasks like the reading-span task described above. It has been found that the amount of trade-off depends on the similarity of the information to be remembered and the information to be processed. For example, remembering numbers while processing spatial information, or remembering spatial information while processing numbers, impair each other much less than when material of the same kind must be remembered and processed.[48] Also, remembering words and processing digits, or remembering digits and processing words, is easier than remembering and processing materials of the same category.[49] These findings are also difficult to explain for the decay hypothesis, because decay of memory representations should depend only on how long the processing task delays rehearsal or recall, not on the content of the processing task. A further problem for the decay hypothesis comes from experiments in which the recall of a list of letters was delayed, either by instructing participants to recall at a slower pace, or by instructing them to say an irrelevant word once or three times in between recall of each letter. Delaying recall had virtually no effect on recall accuracy.[50][51] The interference theory seems to fare best with explaining why the similarity between memory contents and the contents of concurrent processing tasks affects how much they impair each other. More similar materials are more likely to be confused, leading to retrieval competition.
발달[편집]
작업기억의 용량은 유년기에 점진적으로 증가하며[52] 노년기에 점진적으로 감소한다.[53]
유년[편집]
Measures of performance on tests of working memory increase continuously between early childhood and adolescence, while the structure of correlations between different tests remains largely constant.[52] Starting with work in the Neo-Piagetian tradition,[54][55] theorists have argued that the growth of working-memory capacity is a major driving force of cognitive development. This hypothesis has received substantial empirical support from studies showing that the capacity of working memory is a strong predictor of cognitive abilities in childhood.[56] Particularly strong evidence for a role of working memory for development comes from a longitudinal study showing that working-memory capacity at one age predicts reasoning ability at a later age.[57] Studies in the Neo-Piagetian tradition have added to this picture by analyzing the complexity of cognitive tasks in terms of the number of items or relations that have to be considered simultaneously for a solution. Across a broad range of tasks, children manage task versions of the same level of complexity at about the same age, consistent with the view that working memory capacity limits the complexity they can handle at a given age.[58]
노화[편집]
Working memory is among the cognitive functions most sensitive to decline in old age.[59][60] Several explanations have been offered for this decline in psychology. One is the processing speed theory of cognitive aging by Tim Salthouse.[61] Drawing on the finding of general slowing of cognitive processes as people grow older, Salthouse argues that slower processing leaves more time for working-memory contents to decay, thus reducing effective capacity. However, the decline of working-memory capacity cannot be entirely attributed to slowing because capacity declines more in old age than speed.[60][62] Another proposal is the inhibition hypothesis advanced by Lynn Hasher and Rose Zacks.[63] This theory assumes a general deficit in old age in the ability to inhibit irrelevant, or no-longer relevant, information. Therefore, working memory tends to be cluttered with irrelevant contents that reduce the effective capacity for relevant content. The assumption of an inhibition deficit in old age has received much empirical support[64] but, so far, it is not clear whether the decline in inhibitory ability fully explains the decline of working-memory capacity. An explanation on the neural level of the decline of working memory and other cognitive functions in old age has been proposed by West.[65] He argued that working memory depends to a large degree on the pre-frontal cortex, which deteriorates more than other brain regions as we grow old.
훈련[편집]
Torkel Klingberg was the first to investigate whether intensive training of working memory has beneficial effects on other cognitive functions. His pioneering study suggested that working memory can be improved by training in ADHD patients through computerized programs.[66] This study has found that a period of working memory training increases a range of cognitive abilities and increases IQ test scores. Another study of the same group[67] has shown that, after training, measured brain activity related to working memory increased in the prefrontal cortex, an area that many researchers have associated with working memory functions. It has been shown in one study that working memory training increases the density of prefrontal and parietal dopamine receptors (specifically, DRD1) in test persons.[68] However, subsequent work with the same training program has failed to replicate the beneficial effects of training on cognitive performance. A meta-analytic summary of research with Klingberg's training program up to 2011 shows that this training has at best a negligible effect on tests of intelligence and of attention[69]
In another influential study, training with a working memory task (the dual n-back task) has improved performance on a fluid intelligence test in healthy young adults.[70] The improvement of fluid intelligence by training with the n-back task was replicated in 2010,[71] but two studies published in 2012 failed to reproduce the effect.[72][73] The combined evidence from about 30 experimental studies on the effectiveness of working-memory training has been evaluated by several meta-analyses.[74][75] The authors of these meta-analyses disagree in their conclusions as to whether or not working-memory training improves intelligence. Yet, these meta-analyses agree in their estimate of the size of the effect of working-memory training: If there is such an effect, it is likely to be small.
In the brain뇌 속에서[편집]
Neural mechanisms of maintaining information정보를 보유하는 신경상의 기제[편집]
신경적인, 신경전달물질의 기초에 대한 첫 번째 통찰은 동물 연구를 통해 얻어냈다. 1930년대의 야콥센과 풀턴의 연구[76]는 우선 and Fulton in the 1930s first showed that lesions to the PFC impaired spatial working memory performance in monkeys. 원숭이의 두뇌에서 공간적 작업 기억 수행을 저해하는 병변을 보여주었다.The later work of Joaquin Fuster[77] recorded the electrical activity of neurons in the PFC of monkeys while they were doing a delayed matching task. In that task, the monkey sees how the experimenter places a bit of food under one of two identical-looking cups. A shutter is then lowered for a variable delay period, screening off the cups from the monkey's view. After the delay, the shutter opens and the monkey is allowed to retrieve the food from under the cups. Successful retrieval in the first attempt – something the animal can achieve after some training on the task – requires holding the location of the food in memory over the delay period. Fuster found neurons in the PFC that fired mostly during the delay period, suggesting that they were involved in representing the food location while it was invisible. Later research has shown similar delay-active neurons also in the posterior parietal cortex, the thalamus, the caudate, and the globus pallidus.[78] The work of Goldman-Rakic and others showed that principal sulcal, dorsolateral PFC interconnects with all of these brain regions, and that neuronal microcircuits within PFC are able to maintain information in working memory through recurrent excitatory glutamate networks of pyramidal cells that continue to fire throughout the delay period.[79] These circuits are tuned by lateral inhibition from GABAergic interneurons.[80] The neuromodulatory arousal systems markedly alter PFC working memory function; for example, either too little or too much dopamine or norepinephrine impairs PFC network firing[81] and working memory performance.[82]
The research described above on persistent firing of certain neurons in the delay period of working memory tasks shows that the brain has a mechanism of keeping representations active without external input. Keeping representations active, however, is not enough if the task demands maintaining more than one chunk of information. In addition, the components and features of each chunk must be bound together to prevent them from being mixed up. For example, if a red triangle and a green square must be remembered at the same time, one must make sure that "red" is bound to "triangle" and "green" is bound to "square". One way of establishing such bindings is by having the neurons that represent features of the same chunk fire in synchrony, and those that represent features belonging to different chunks fire out of sync.[83] In the example, neurons representing redness would fire in synchrony with neurons representing the triangular shape, but out of sync with those representing the square shape. So far, there is no direct evidence that working memory uses this binding mechanism, and other mechanisms have been proposed as well.[84] It has been speculated that synchronous firing of neurons involved in working memory oscillate with frequencies in the theta band (4 to 8 Hz). Indeed, the power of theta frequency in the EEG increases with working memory load,[85] and oscillations in the theta band measured over different parts of the skull become more coordinated when the person tries to remember the binding between two components of information.[86]
뇌의 국부성[편집]
Localization of brain functions in humans has become much easier with the advent of brain imaging methods (PET and fMRI). This research has confirmed that areas in the PFC are involved in working memory functions. During the 1990s much debate has centered on the different functions of the ventrolateral (i.e., lower areas) and the dorsolateral (higher) areas of the PFC. A human lesion study provides additional evidence for the role of the dorsolateral prefrontal cortex in working memory.[87] One view was that the dorsolateral areas are responsible for spatial working memory and the ventrolateral areas for non-spatial working memory. Another view proposed a functional distinction, arguing that ventrolateral areas are mostly involved in pure maintenance of information, whereas dorsolateral areas are more involved in tasks requiring some processing of the memorized material. The debate is not entirely resolved but most of the evidence supports the functional distinction.[88]
Brain imaging has also revealed that working memory functions are not limited to the PFC. A review of numerous studies[89] shows areas of activation during working memory tasks scattered over a large part of the cortex. There is a tendency for spatial tasks to recruit more right-hemisphere areas, and for verbal and object working memory to recruit more left-hemisphere areas. The activation during verbal working memory tasks can be broken down into one component reflecting maintenance, in the left posterior parietal cortex, and a component reflecting subvocal rehearsal, in the left frontal cortex (Broca's area, known to be involved in speech production).[90]
There is an emerging consensus that most working memory tasks recruit a network of PFC and parietal areas. A study has shown that during a working memory task the connectivity between these areas increases.[91] Another study has demonstrated that these areas are necessary for working memory, and not simply activated accidentally during working memory tasks, by temporarily blocking them through transcranial magnetic stimulation (TMS), thereby producing an impairment in task performance.[92]
A current debate concerns the function of these brain areas. The PFC has been found to be active in a variety of tasks that require executive functions.[33] This has led some researchers to argue that the role of PFC in working memory is in controlling attention, selecting strategies, and manipulating information in working memory, but not in maintenance of information. The maintenance function is attributed to more posterior areas of the brain, including the parietal cortex.[93][94] Other authors interpret the activity in parietal cortex as reflecting executive functions, because the same area is also activated in other tasks requiring attention but not memory.[95]
A 2003 meta-analysis of 60 neuroimaging studies found left frontal cortex was involved in low-task demand verbal working memory and right frontal cortex for spatial working memory. Brodmann's areas (BAs) 6, 8, and 9, in the superior frontal cortex was involved when working memory must be continuously updated and when memory for temporal order had to be maintained. Right Brodmann 10 and 47 in the ventral frontal cortex were involved more frequently with demand for manipulation such as dual-task requirements or mental operations, and Brodmann 7 in the posterior parietal cortex was also involved in all types of executive function.[96]
Working memory has been suggested to involve two processes with different neuroanatomical locations in the frontal and parietal lobes.[97] First, a selection operation that retrieves the most relevant item, and second an updating operation that changes the focus of attention made upon it. Updating the attentional focus has been found to involve the transient activation in the caudal superior frontal sulcus and posterior parietal cortex, while increasing demands on selection selectively changes activation in the rostral superior frontal sulcus and posterior cingulate/precuneus.[97]
Articulating the differential function of brain regions involved in working memory is dependent on tasks able to distinguish these functions.[98] Most brain imaging studies of working memory have used recognition tasks such as delayed recognition of one or several stimuli, or the n-back task, in which each new stimulus in a long series must be compared to the one presented n steps back in the series. The advantage of recognition tasks is that they require minimal movement (just pressing one of two keys), making fixation of the head in the scanner easier. Experimental research and research on individual differences in working memory, however, has used largely recall tasks (e.g., the reading span task, see below). It is not clear to what degree recognition and recall tasks reflect the same processes and the same capacity limitations.
Brain imaging studies have been conducted with the reading span task or related tasks. Increased activation during these tasks was found in the PFC and, in several studies, also in the anterior cingulate cortex (ACC). People performing better on the task showed larger increase of activation in these areas, and their activation was correlated more over time, suggesting that their neural activity in these two areas was better coordinated, possibly due to stronger connectivity.[99][100]
신경 모델[편집]
One approach to model the neurophysiology and the functioning of working memory is the prefrontal cortex basal ganglia working memory (PBWM).
Effects of stress on neurophysiology스트레스의 신경생리학적 효과[편집]
Working memory is impaired by acute and chronic psychological stress. This phenomenon was first discovered in animal studies by Arnsten and colleagues,[101] who have shown that stress-induced catecholamine release in PFC rapidly decreases PFC neuronal firing and impairs working memory performance through feedforward, intracellular signaling pathways.[102] Exposure to chronic stress leads to more profound working memory deficits and additional architectural changes in PFC, including dendritic atrophy and spine loss,[103] which can be prevented by inhibition of protein kinase C signaling.[104] fMRI research has extended this research to humans, and confirms that reduced working memory caused by acute stress links to reduced activation of the PFC, and stress increased levels of catecholamines.[105] Imaging studies of medical students undergoing stressful exams have also shown weakened PFC functional connectivity, consistent with the animal studies.[106] The marked effects of stress on PFC structure and function may help to explain how stress can cause or exacerbate mental illness. The more stress in one's life, the lower the efficiency of working memory in performing simple cognitive tasks. Students who performed exercises that reduced the intrusion of negative thoughts showed an increase in their working memory capacity. Mood states (positive or negative) can have an influence on the neurotransmitter dopamine, which in turn can affect problem solving.[107]
Effects of alcohol on neurophysiology알코올의 신경생리학적 효과[편집]
Alcohol abuse can result in brain damage which impairs working memory.[108] Alcohol has an effect on the blood-oxygen-level-dependent (BOLD) response. The BOLD response correlates increased blood oxygenation with brain activity, which makes this response a useful tool for measuring neuronal activity.[109] The BOLD response affects regions of the brain such as the basal ganglia and thalamus when performing a working memory task. Adolescents who start drinking at a young age show a decreased BOLD response in these brain regions.[110] Alcohol dependent young women in particular exhibit less of a BOLD response in parietal and frontal cortices when performing a spatial working memory task.[111] Binge drinking, specifically, can also affect one's performance on working memory tasks, particularly visual working memory.[112][113] Additionally, there seems to be a gender difference in regards to how alcohol affects working memory. While women perform better on verbal working memory tasks after consuming alcohol compared to men, they appear to perform worse on spatial working memory tasks as indicated by less brain activity.[114][115] Finally, age seems to be an additional factor. Older adults are more susceptible than others to the effects of alcohol on working memory.[116]
Genetics유전학[편집]
Behavioral genetics행동유전학[편집]
Individual differences in working-memory capacity are to some extent heritable; that is, about half of the variation between individuals is related to differences in their genes.[117][118][119] The genetic component of variability of working-memory capacity is largely shared with that of fluid intelligence.[118][117]
개별 유전자를 식별하는 시도[편집]
Little is known about which genes are related to the functioning of working memory. Within the theoretical framework of the multi-component model, one candidate gene has been proposed, namely ROBO1 for the hypothetical phonological loop component of working memory.[120]
Role in academic achievement학문적 성취에서의 역할[편집]
Working memory capacity is correlated with learning outcomes in literacy and numeracy. Initial evidence for this relation comes from the correlation between working-memory capacity and reading comprehension, as first observed by Daneman and Carpenter (1980)[121] and confirmed in a later meta-analytic review of several studies.[122] Subsequent work found that working memory performance in primary school children accurately predicted performance in mathematical problem solving.[123] One longitudinal study showed that a child's working memory at 5 years old is a better predictor of academic success than IQ.[124]
In a large-scale screening study, one in ten children in mainstream classrooms were identified with working memory deficits. The majority of them performed very poorly in academic achievements, independent of their IQ.[125] Similarly, working memory deficits have been identified in national curriculum low-achievers as young as seven years of age.[126] Without appropriate intervention, these children lag behind their peers. A recent study of 37 school-age children with significant learning disabilities has shown that working memory capacity at baseline measurement, but not IQ, predicts learning outcomes two years later.[127] This suggests that working memory impairments are associated with low learning outcomes and constitute a high risk factor for educational underachievement for children. In children with learning disabilities such as dyslexia, ADHD, and developmental coordination disorder, a similar pattern is evident.[128][129][130][131]
Relation to attention주의와의 관계[편집]
There is some evidence that optimal working memory performance links to the neural ability to focus attention on task-relevant information and to ignore distractions,[132] and that practice-related improvement in working memory is due to increasing these abilities.[133] One line of research suggests a link between the working memory capacities of a person and their ability to control the orientation of attention to stimuli in the environment.[134] Such control enables people to attend to information important for their current goals, and to ignore goal-irrelevant stimuli that tend to capture their attention due to their sensory saliency (such as an ambulance siren). The direction of attention according to one's goals is assumed to rely on "top-down" signals from the pre-frontal cortex (PFC) that biases processing in posterior cortical areas.[135] Capture of attention by salient stimuli is assumed to be driven by "bottom-up" signals from subcortical structures and the primary sensory cortices.[136] The ability to override "bottom-up" capture of attention differs between individuals, and this difference has been found to correlate with their performance in a working-memory test for visual information.[134] Another study, however, found no correlation between the ability to override attentional capture and measures of more general working-memory capacity.[137]
Relationship with neural disorders신경 장애와의 관계[편집]
An impairment of working memory functioning is normally seen in several neural disorders:
ADHD: Several authors[138] have proposed that symptoms of ADHD arise from a primary deficit in a specific executive function (EF) domain such as working memory, response inhibition or a more general weakness in executive control.[139] A meta-analytical review cites several studies that found significant lower group results for ADHD in spatial and verbal working memory tasks, and in several other EF tasks. However, the authors concluded that EF weaknesses neither are necessary nor sufficient to cause all cases of ADHD.[139]
Several neurotransmitters, such as dopamine and glutamate may be both involved in ADHD and working memory. Both are associated with the frontal brain, self-direction and self-regulation, but cause–effect have not been confirmed, so it is unclear whether working memory dysfunction leads to ADHD, or ADHD distractibility leads to poor functionality of working memory, or if there is some other connection.[140][141][142]
파킨슨병: Patients with Parkinson's show signs of a reduced verbal function of working memory. They wanted to find if the reduction is due to a lack of ability to focus on relevant tasks, or a low amount of memory capacity. Twenty-one patients with Parkinson's were tested in comparison to the control group of 28 participants of the same age.The researchers found that both hypotheses were the reason working memory function is reduced which did not fully agree with their hypothesis that it is either one or the other.[143]
알츠하이머: As Alzheimer's disease becomes more serious, less working memory functions. There is one study that focuses on the neural connections and fluidity of working memory in mice brains. Half of the mice were given an injection that is similar to Alzheimer's effects, and the other half were not. Then they were expected to go through a maze that is a task to test working memory. The study help answer questions about how Alzheimer's can deteriorate the working memory and ultimately obliterate memory functions.[144]
헌팅턴무도병: A group of researchers hosted a study that researched the function and connectivity of working memory over a 30-month longitudinal experiment. It found that there were certain places in the brain where most connectivity was decreased in pre-Huntington diseased patients, in comparison to the control group that remained consistently functional.[145]
참고 문서[편집]
- Atkinson–Shiffrin memory model
- 스크립트 오류: "Section link" 모듈이 없습니다.
- Autism and working memory
- Fuzzy-trace theory
- Intermediate-term memory
- Memory and aging
- Prefrontal cortex basal ganglia working memory (PBWM)
- Cognitive architecture
- Tim Shallice
각주[편집]
- ↑ 스크립트 오류: "citation/CS1" 모듈이 없습니다.
- ↑ 2.0 2.1 스크립트 오류: "Citation/CS1" 모듈이 없습니다.
- ↑ 스크립트 오류: "citation/CS1" 모듈이 없습니다.
- ↑ 스크립트 오류: "Citation/CS1" 모듈이 없습니다.
- ↑ 스크립트 오류: "citation/CS1" 모듈이 없습니다.
- ↑ 스크립트 오류: "Citation/CS1" 모듈이 없습니다.
- ↑ 스크립트 오류: "citation/CS1" 모듈이 없습니다.
- ↑ 스크립트 오류: "citation/CS1" 모듈이 없습니다.[쪽 번호 필요]
- ↑ 9.0 9.1 스크립트 오류: "citation/CS1" 모듈이 없습니다.
- ↑ 스크립트 오류: "citation/CS1" 모듈이 없습니다.
- ↑ 스크립트 오류: "citation/CS1" 모듈이 없습니다.
- ↑ 스크립트 오류: "citation/CS1" 모듈이 없습니다.
- ↑ 스크립트 오류: "citation/CS1" 모듈이 없습니다.
- ↑ 스크립트 오류: "citation/CS1" 모듈이 없습니다.
- ↑ 스크립트 오류: "Citation/CS1" 모듈이 없습니다.
- ↑ 스크립트 오류: "Citation/CS1" 모듈이 없습니다.
- ↑ 스크립트 오류: "citation/CS1" 모듈이 없습니다.[쪽 번호 필요]
- ↑ 스크립트 오류: "Citation/CS1" 모듈이 없습니다.
- ↑ 스크립트 오류: "Citation/CS1" 모듈이 없습니다.
- ↑ 스크립트 오류: "Citation/CS1" 모듈이 없습니다. Republished: 스크립트 오류: "Citation/CS1" 모듈이 없습니다.
- ↑ 스크립트 오류: "Citation/CS1" 모듈이 없습니다.
- ↑ 스크립트 오류: "Citation/CS1" 모듈이 없습니다.
- ↑ 스크립트 오류: "Citation/CS1" 모듈이 없습니다.
- ↑ 스크립트 오류: "Citation/CS1" 모듈이 없습니다.
- ↑ 스크립트 오류: "Citation/CS1" 모듈이 없습니다.
- ↑ 스크립트 오류: "Citation/CS1" 모듈이 없습니다.
- ↑ 스크립트 오류: "Citation/CS1" 모듈이 없습니다.
- ↑ 스크립트 오류: "Citation/CS1" 모듈이 없습니다.
- ↑ 스크립트 오류: "Citation/CS1" 모듈이 없습니다.
- ↑ 스크립트 오류: "Citation/CS1" 모듈이 없습니다.
- ↑ 스크립트 오류: "Citation/CS1" 모듈이 없습니다.
- ↑ 스크립트 오류: "Citation/CS1" 모듈이 없습니다.
- ↑ 33.0 33.1 스크립트 오류: "Citation/CS1" 모듈이 없습니다.
- ↑ 스크립트 오류: "Citation/CS1" 모듈이 없습니다.
- ↑ 35.0 35.1 스크립트 오류: "Citation/CS1" 모듈이 없습니다.
- ↑ 스크립트 오류: "Citation/CS1" 모듈이 없습니다.
- ↑ 스크립트 오류: "Citation/CS1" 모듈이 없습니다.
- ↑ 스크립트 오류: "Citation/CS1" 모듈이 없습니다.
- ↑ 스크립트 오류: "Citation/CS1" 모듈이 없습니다.
- ↑ 스크립트 오류: "citation/CS1" 모듈이 없습니다.
- ↑ 스크립트 오류: "Citation/CS1" 모듈이 없습니다.
- ↑ 스크립트 오류: "citation/CS1" 모듈이 없습니다.
- ↑ 스크립트 오류: "Citation/CS1" 모듈이 없습니다.
- ↑ 스크립트 오류: "Citation/CS1" 모듈이 없습니다.
- ↑ 스크립트 오류: "Citation/CS1" 모듈이 없습니다.
- ↑ 스크립트 오류: "Citation/CS1" 모듈이 없습니다.
- ↑ 스크립트 오류: "Citation/CS1" 모듈이 없습니다.
- ↑ 스크립트 오류: "Citation/CS1" 모듈이 없습니다.
- ↑ 스크립트 오류: "Citation/CS1" 모듈이 없습니다.
- ↑ 스크립트 오류: "Citation/CS1" 모듈이 없습니다.
- ↑ 스크립트 오류: "Citation/CS1" 모듈이 없습니다.
- ↑ 52.0 52.1 스크립트 오류: "Citation/CS1" 모듈이 없습니다.
- ↑ 스크립트 오류: "Citation/CS1" 모듈이 없습니다.
- ↑ 스크립트 오류: "Citation/CS1" 모듈이 없습니다.
- ↑ Case, R. (1985). Intellectual development. Birth to adulthood. New York: Academic Press.
- ↑ Jarrold, C., & Bayliss, D. M. (2007). Variation in working memory due to typical and atypical development. In A. R. A. Conway, C. Jarrold, M. J. Kane, A. Miyake & J. N. Towse (Eds.), Variation in working memory (pp. 137–161). New York: Oxford University Press.
- ↑ 스크립트 오류: "Citation/CS1" 모듈이 없습니다.
- ↑ 스크립트 오류: "Citation/CS1" 모듈이 없습니다.
- ↑ 스크립트 오류: "Citation/CS1" 모듈이 없습니다.
- ↑ 60.0 60.1 스크립트 오류: "Citation/CS1" 모듈이 없습니다.
- ↑ 스크립트 오류: "Citation/CS1" 모듈이 없습니다.
- ↑ 스크립트 오류: "Citation/CS1" 모듈이 없습니다.
- ↑ Hasher, L., & Zacks, R. T. (1988). Working memory, comprehension, and aging: A review and new view. In G. H. Bower (Ed.), The psychology of learning and motivation, Vol. 22, (pp. 193–225). New York: Academic Press.
- ↑ Hasher, L., Zacks, R. T., & May, C. P. (1999). Inhibitory control, circadian arousal, and age. In D. Gopher & A. Koriat (Eds.), Attention and Performance (pp. 653–675). Cambridge, MA: MIT Press.
- ↑ 스크립트 오류: "Citation/CS1" 모듈이 없습니다.
- ↑ 스크립트 오류: "Citation/CS1" 모듈이 없습니다.
- ↑ 스크립트 오류: "Citation/CS1" 모듈이 없습니다.
- ↑ 스크립트 오류: "Citation/CS1" 모듈이 없습니다.
- ↑ 스크립트 오류: "Citation/CS1" 모듈이 없습니다.
- ↑ 스크립트 오류: "Citation/CS1" 모듈이 없습니다.
- ↑ 스크립트 오류: "Citation/CS1" 모듈이 없습니다.
- ↑ 스크립트 오류: "Citation/CS1" 모듈이 없습니다.
- ↑ 스크립트 오류: "Citation/CS1" 모듈이 없습니다.
- ↑ 스크립트 오류: "Citation/CS1" 모듈이 없습니다.
- ↑ 스크립트 오류: "Citation/CS1" 모듈이 없습니다.
- ↑ 스크립트 오류: "Citation/CS1" 모듈이 없습니다.
- ↑ 스크립트 오류: "Citation/CS1" 모듈이 없습니다.
- ↑ 스크립트 오류: "Citation/CS1" 모듈이 없습니다.
- ↑ 스크립트 오류: "Citation/CS1" 모듈이 없습니다.
- ↑ 스크립트 오류: "Citation/CS1" 모듈이 없습니다.
- ↑ 스크립트 오류: "Citation/CS1" 모듈이 없습니다.
- ↑ 스크립트 오류: "Citation/CS1" 모듈이 없습니다.
- ↑ 스크립트 오류: "Citation/CS1" 모듈이 없습니다.
- ↑ 스크립트 오류: "citation/CS1" 모듈이 없습니다.
- ↑ 스크립트 오류: "citation/CS1" 모듈이 없습니다.
- ↑ 스크립트 오류: "Citation/CS1" 모듈이 없습니다.
- ↑ 스크립트 오류: "Citation/CS1" 모듈이 없습니다.
- ↑ 스크립트 오류: "Citation/CS1" 모듈이 없습니다.
- ↑ 스크립트 오류: "Citation/CS1" 모듈이 없습니다.
- ↑ 스크립트 오류: "Citation/CS1" 모듈이 없습니다.
- ↑ 스크립트 오류: "Citation/CS1" 모듈이 없습니다.
- ↑ 스크립트 오류: "Citation/CS1" 모듈이 없습니다.
- ↑ 스크립트 오류: "Citation/CS1" 모듈이 없습니다.
- ↑ 스크립트 오류: "Citation/CS1" 모듈이 없습니다.
- ↑ 스크립트 오류: "Citation/CS1" 모듈이 없습니다.
- ↑ 스크립트 오류: "Citation/CS1" 모듈이 없습니다.
- ↑ 97.0 97.1 스크립트 오류: "Citation/CS1" 모듈이 없습니다.
- ↑ 스크립트 오류: "Citation/CS1" 모듈이 없습니다.
- ↑ 스크립트 오류: "Citation/CS1" 모듈이 없습니다.
- ↑ 스크립트 오류: "Citation/CS1" 모듈이 없습니다.
- ↑ 스크립트 오류: "Citation/CS1" 모듈이 없습니다.
- ↑ 스크립트 오류: "Citation/CS1" 모듈이 없습니다.
- ↑ 스크립트 오류: "Citation/CS1" 모듈이 없습니다.
- ↑ 스크립트 오류: "Citation/CS1" 모듈이 없습니다.
- ↑ 스크립트 오류: "Citation/CS1" 모듈이 없습니다.
- ↑ 스크립트 오류: "Citation/CS1" 모듈이 없습니다.
- ↑ 스크립트 오류: "citation/CS1" 모듈이 없습니다.
- ↑ 스크립트 오류: "Citation/CS1" 모듈이 없습니다.
- ↑ 스크립트 오류: "Citation/CS1" 모듈이 없습니다.
- ↑ 스크립트 오류: "Citation/CS1" 모듈이 없습니다.
- ↑ 스크립트 오류: "Citation/CS1" 모듈이 없습니다.
- ↑ 스크립트 오류: "Citation/CS1" 모듈이 없습니다.
- ↑ 스크립트 오류: "Citation/CS1" 모듈이 없습니다.
- ↑ 스크립트 오류: "Citation/CS1" 모듈이 없습니다.
- ↑ 스크립트 오류: "Citation/CS1" 모듈이 없습니다.
- ↑ 스크립트 오류: "Citation/CS1" 모듈이 없습니다.
- ↑ 117.0 117.1 스크립트 오류: "Citation/CS1" 모듈이 없습니다.
- ↑ 118.0 118.1 스크립트 오류: "Citation/CS1" 모듈이 없습니다.
- ↑ 스크립트 오류: "Citation/CS1" 모듈이 없습니다.
- ↑ 스크립트 오류: "Citation/CS1" 모듈이 없습니다.
- ↑ 스크립트 오류: "Citation/CS1" 모듈이 없습니다.
- ↑ 스크립트 오류: "Citation/CS1" 모듈이 없습니다.
- ↑ 스크립트 오류: "Citation/CS1" 모듈이 없습니다.
- ↑ 스크립트 오류: "Citation/CS1" 모듈이 없습니다.
- ↑ 스크립트 오류: "Citation/CS1" 모듈이 없습니다.
- ↑ 스크립트 오류: "Citation/CS1" 모듈이 없습니다.
- ↑ 스크립트 오류: "Citation/CS1" 모듈이 없습니다.
- ↑ 스크립트 오류: "citation/CS1" 모듈이 없습니다.
- ↑ 스크립트 오류: "citation/CS1" 모듈이 없습니다.
- ↑ 스크립트 오류: "citation/CS1" 모듈이 없습니다.
- ↑ 스크립트 오류: "citation/CS1" 모듈이 없습니다.
- ↑ 스크립트 오류: "Citation/CS1" 모듈이 없습니다.
- ↑ 스크립트 오류: "Citation/CS1" 모듈이 없습니다.
- ↑ 134.0 134.1 스크립트 오류: "Citation/CS1" 모듈이 없습니다.
- ↑ 스크립트 오류: "Citation/CS1" 모듈이 없습니다.
- ↑ 스크립트 오류: "Citation/CS1" 모듈이 없습니다.
- ↑ 스크립트 오류: "Citation/CS1" 모듈이 없습니다.
- ↑ Barkley; Castellanos and Tannock; Pennington and Ozonoff; Schachar (according to the source)
- ↑ 139.0 139.1 스크립트 오류: "Citation/CS1" 모듈이 없습니다.
- ↑ Working Memory as a Core Deficit in ADHD: Preliminary Findings and Implications – 2008
- ↑ 스크립트 오류: "Citation/CS1" 모듈이 없습니다.
- ↑ 스크립트 오류: "Citation/CS1" 모듈이 없습니다.
- ↑ 스크립트 오류: "Citation/CS1" 모듈이 없습니다.
- ↑ 스크립트 오류: "Citation/CS1" 모듈이 없습니다.
- ↑ 스크립트 오류: "Citation/CS1" 모듈이 없습니다.
외부 링크[편집]
- Models of Working Memory (Mechanisms of Active Maintenance and Executive Control)
- Peter Doolittle: How your "working memory" makes sense of the world, TED Talk, published November 22, 2013.
This article "작업기억" is from Wikipedia. The list of its authors can be seen in its historical and/or the page Edithistory:작업기억. Articles copied from Draft Namespace on Wikipedia could be seen on the Draft Namespace of Wikipedia and not main one.